A New Contender for the Theory of Everything
For more than 100 years, physicists have been trying to unite the two great theories of the 20th century — general relativity and quantum mechanics — that together seek to explain the nature of reality at scales from the galactic down to the subatomic. A so-called theory of everything, or unified theory, would account for all matter and force, and explain the fundamental structure of space and time, leading to a complete understanding of the universe. So far, the search has been elusive. Gravity, in particular, has proved troublesome to account for at a quantum level.
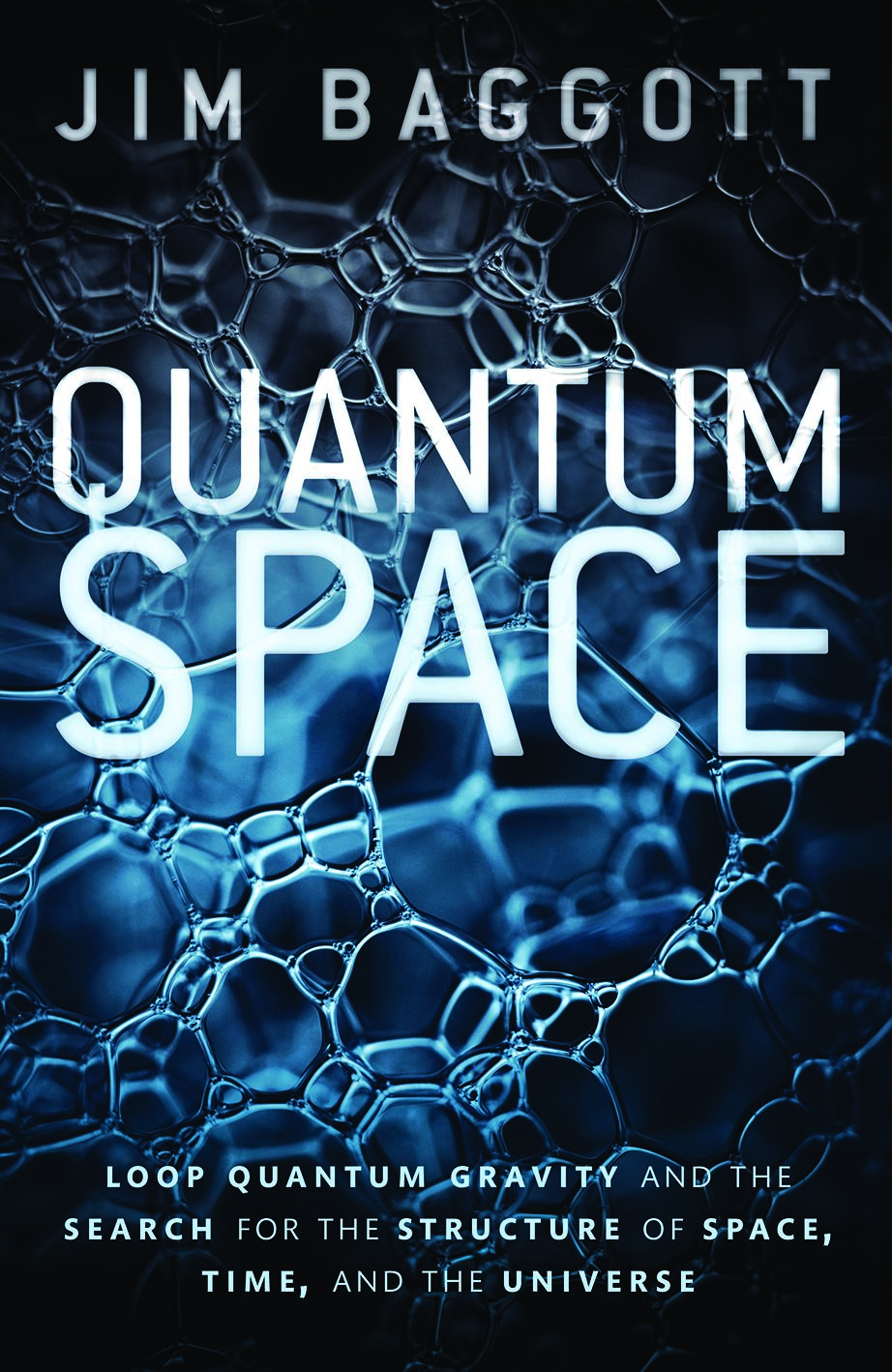
Unlike general relativity, the author says, a “quantum theory of gravity means that there’s ultimately a density you can’t go beyond.”
The most popular contender over the past few decades has been string theory, and the related concepts of superstring theory and M-theory, in which particles are considered as tiny units of one-dimensional string. However, a lesser-known theory has also gained traction; loop quantum gravity (LQG), which attempts to solve the quantum gravity problem by focusing on the very fabric of spacetime, rather than the particles themselves.
In “Quantum Space,” the popular-science writer Jim Baggott lays out the basic principles of LQG for science enthusiasts. The book looks at how loop quantum gravity has emerged by following the work of two of its leading proponents, Carlo Rovelli and Lee Smolin, and assesses where the theory is now, and where it might be going.
Although the concepts are — not surprisingly — mind-boggling, Baggott asks deep questions about the nature of the universe, what space is actually composed of, and the existence of time itself. (The book covers a lot of challenging material, however, and some prior reading may help readers find their way.)
For this installment of the Undark Five, I spoke with Baggott about the book and some of the ideas behind loop quantum gravity. Our exchange has been edited and condensed for space and clarity.
Undark: Why did you write this book and why now?
Jim Baggott: So, over the years I’ve been reading quite a bit about superstring theory, and some of the ever-expanding list of assumptions that needed to be invoked in order to make sense of anything string theory- related. I eventually got a bit concerned that people might start to think that string theory was an accepted science — which I believe starts to strain the credibility of scientists much more broadly.
Then it began to occur to me that there hadn’t been enough published recently on loop quantum gravity— the main alternative to string theory in the search for a unified theory. I thought actually the time is possibly right for a counter to all of the great PR on super string theory and the multiverse. By actually going into some detail on what LQG is, where it came from, what problems it’s trying to address. You still have to accept a lot of assumptions for LQG, but not as many as string theory, I think.
UD: What is loop quantum gravity, and how is it different from string theory?
JB: Loop quantum gravity is an alternative to string theory, which in its earliest formulation says that particles are composed of one-dimensional lines or strings of energy. Different particles, in this theory, represent different vibrational patterns in these strings. String theory got complicated very quickly with suggestions of 26 different dimensions. Then things calmed down with the introduction of supersymmetry, which enabled the number of dimensions to be reduced to six, in addition to the three we usually refer to, plus time.
LQG is an approach that says to start with general relativity — the theory that relates gravity to the curvature of space and time — and then let’s try to find a way to “quantize” it — that is, to change continuous variables into those which assume only certain discrete magnitudes. Essentially, the theory suggests that the ultimate essence of space is comprised of little loops of gravitational force. Then, following this formulation to its logical conclusion, one finds that there is an ultimate unit of volume — the Planck scale — which cannot be further divided.
So unlike general relativity, which allows for the possibility of a singularity — things being squeezed down to an infinite density — a quantum theory of gravity means that there’s ultimately a density you can’t go beyond.
UD: In a chapter called “To get there I wouldn’t start from here,’’ you write that “the standard model of particle physics is a collection of quantum field theories, which although they meet the demands of special relativity, still assume a background spacetime’’ — i.e. a set spacetime curved by matter in which everything takes place. What is wrong with our current understanding of physics in this sense?
JB: There’s nothing really wrong with it. The standard model of particle physics works incredibly well — as shown by the recent discovery of the Higgs Boson. But it is a fact that quantum mechanics, when it was conceived in the 1920s, carried over the structure of spacetime that had been used as the background for classical physics. So although things like energy and matter were quantized during that shift to quantum mechanics, space and time remained continuous.
In general relativity, space and time emerge as a kind of dynamic variable of the theory — it should not be assumed as a background. Rather, it demonstrates that gravity is not a force, it is the result of the curvature of spacetime caused by the presence of mass.
So, that’s really difficult, if you’re trying to bring together these two theoretical structures and one has a very different view of what spacetime is about and where it comes from. The idea was to try and find a way to make spacetime emergent in the field of quantum gravity.
UD: What does quantum gravity tell us about the existence of time, or the nonexistence of time?
JB: The great physicist Paul Dirac found fairly early on that you kind of lose track of time in quantum mechanics. Time gets lost in the equations, so you have to bring it back. It has to be reinstated, in effect, as a juxtaposition of different spatial states, i.e. different geometries. So, as the geometry changes, it creates the impression, or illusion, of time.
Talk to co-founder of the LQG theory, Carlo Rovelli, and he will tell you that time as we perceive it is an illusion, and that when you get down to the Planck scale, time ceases to exist at all. When you recreate time in LQG it’s through something called a spin foam — which means that as you juxtapose all of this geometry in space it becomes a recreation of the flow of time. But is this something that truly reflects the real nature of time? Honestly, who knows?
UD: Where do you think the theory is moving now? Can observations and experimentation help to prove it?
JB: This is a really difficult area. From a theoretical point of view, the ideas behind LQG are taking place at a scale you’ll never be able to get to. The scale at which all this takes place — known as the Planck scale — is so much smaller than subatomic particles and you’ll never be able to build a collider that gets down to that level. So, if we can’t build a collider big enough, we need to look at the universe and see how observational cosmology can give us some clues about whether we’re on the right track or not.
This brings us into the field of loop quantum cosmology, which is a large-scale picture of the universe based on LQG. Loop quantum cosmology suggests that we may have had a Big Bounce — the universe collapsed in on itself at one stage and bounced back out — rather than a Big Bang where the universe exploded from nothing. There’s a suggestion that when the technology (large-scale gravitational wave detectors) is sufficiently sophisticated and precise, we might be able to find subtle differences between the current Big Bang model and what loop quantum cosmology predicts.
Regarding the future, we’re talking about really large-scale experiments — satellites with detectors in space which cost a huge amount of money and depend on the continuing support of taxpayers for funding. There’s also the hope that something surprising comes up from the Large Hadron Collider or that some other new generation of particle collider sheds some light on the matter. I don’t know what experimental results will ever give us what we’re looking for. I think it’s going to have to be something rather grand-scale, and who knows, may yet still produce some surprises.
Conor Purcell is a science journalist based in Dublin. He is the founding editor of wideorbits.com and can be found on Twitter @ConorPPurcell.
Comments are automatically closed one year after article publication. Archived comments are below.
Everything is conected, through the spiritual energy of human existence and that the very energy of this cannot cease to exist, that is our key to all. Trying to further my theory, but drugs for dimensional exploration are hardha to come by.
At the heart of vibration R 1 and 0 or force no force. These are Quantum entangled, and different patterns make different frequencies. IE somewhere you may have a high frequency cluster of 1 1’S
Good interview.It is elaborate on their views.Please find my comments on their views.
1. LQG can not explain the formation of ‘smooth space time’ with ‘discrete’ quantum particles. So it is not a better idea to synchronize QM with GR .
2. In their view, time is an illusion.But as per me time is also like space and both are exchangeable. I have submitted a paper to the journal ‘Foundations of Physics’ with a proposal for experimental verification . Prof. Carlo Rovelli is chief editor. It was rejected for publication. Please find the link of that preprint of it is for reference: https://doi.org/10.21203/rs.3.rs-40479/v2. It will be supported by two more proofs(next paper on a theory of origin of universe behind the experimental results on Hubble’s constant ‘H’). My proposed results(of my preprint)along with recent experiments on ‘H’ will completely change their notion of space time.
3. Relativity can not be compatible with QM unless otherwise it is modified. A new theory is required to explain ‘absolute velocities'(like light) in frame work of ‘Relativity’. Already I made the calculations for it. But I want to submit it after publication of present papers. This will explain quantum particles in terms of General Relativity and the specialty is without this explanation quantum mechanics can not be a part of General relativity.
4. ‘Time’ is an emerging fact involved from ‘physics of Consciousness’. It is must to synchronize QM with GR.
In my opinion, gravity shouldn’t be ignored however small it may be. Thus will prove that higher mass surely reaches ground before smaller mass. Theory of Everything is possible if we change some statements.
I think that quantum entanglement, particularly spin entanglement, demonstrates that the magnetic force operates almost instantaneously. Similarly for the electric force.
When both forces are entangled, photons are produced that travel at the speed of light. When just one of the two forces act, Quantum Field Theory assumes that virtual photons carry the force.
However, virtual photons may not exist and may merely be a mathematical construct to preserve relativity.
Consider two permanent magnets (or two charges) held in place. They exert a force on each other, but nothing is moving. How are virtual photons generated when nothing is moving?
Why can’t we have relativistic effects, limited by the speed of light, when objects are moving and instantaneous effects when nothing is moving?
When a charge (or magnet), moves back and forth, we may have photons, governed by relativity, emmitted in one direction and instantaneous forces acting in a perpendicular direction.
I think space and time are separate things and it’s matter of convenience that they are together. Hopefully, soon we shall break the speed of light barrier and open up a floodgate of new learnings.
Space may be in astate of non-existance. Time may be in a state of co-dependance. Thus the reality may be that time, and space are codependants. You cannot have one without the other. To exist there has to be a state of non-existance. But in a spiritual world where conciousness niether has to be efected by mass/time/gravity,.. after death the skin is shed, and time becomes relativity. A disembodied soul has the atomic weight of the discrepancy of universal measurement. Outside of space, and time; conciousness can exist unfettered by reality. This is where god comes into play. God; G=center/source, O=whole/entirety, D=participle/way.
It is possible that god was born from nothing(space), and space was simultaneously born from god. Conciousness, and non-conciousness coexisting. So good, and evil(eve ill), knowledge, and ignorance are biproducts of this three dimentional reality between god, reality, and non-reality. Biproducts that moralility must envelope in order to be “saved”.
This is not a choice scientifically speaking, it’s a perogative of survival. Those who choose science, only study god’s creation. They do not, it seems to me, have the nerve to study god itself.
Thanks for reading.
1) Interesting how these discussions always end up involving the Divine…
2) does anyone have any idea whatsoever where all the energy required for all of this comes from? In my experience there’s gotta be fuel for the flame. When the match is burnt, the fire goes out. Done. When the fuel in your lighter is gone you’re bumming a light from your mate. Or buying a new lighter. Where does the vast amount of energy – which must underlay everything to keep reality existent – come from?!
Sorry, but I just can’t hold my smile looking at the comments here. Just one equation please (except of the matter=fabric of cause)? I think most would be surprised…
The Universe is vast & mysterious. There are things we don’t know. There are things we do know. Of the latter, it appears that there is some sort of direction, or order, to the ebbs & flows of energy & matter where ever we explore. The one thing that hasn’t been confirmed is the absense of the Universe’s apparent orderly functioning, despite our human efforts to describe things as happenstance. To really appreciate all there is of this known Universe of ours, it might be judicious to comprehend the Universe’s orderliness before we try looking for neighboring, or parallel, universes.
My Dear Reverend. “orderliness” is a subjective evaluation, where one sees order others see chaos. Orderliness is a an anthropocentric evaluation. I would instead use the word “system”. Noting the behavior of objects in a system is about setting parameters of which observable phenomenon can be experimentally predicted. But system is expandable as its parameters can be defined and refined. Orderliness appeals to a sense of symmetry and the subjective satisfaction that elements belong in the places they are perceived to do. A system can be understood and communicated, order is an emotional response to the perception of a system. But just to head of the unspoken insinuation that for there to be order there must be a someone to design it. A system does not require a designer. As system has not set of values, order does….
The many array of ideas and thoughts that go into the time of man’s quest for the ultimate answer is a fascinating one! The latest ideas,theory s and published remarks and thoughts of what we and it is,sparks only more words and phrases to achieve more convusion! See the answers are in a science that has not been thought or conceived of yet! (Comprehended) The truths of exixtence, reality and the ideas of our exact nature lie in a different state of comprehending, not the thinking!
I’ve been screaming at the top of my lungs on forums for years that you can’t have infinite density. Glad to see that getting some traction. It is a bit confusing, as you change relative velocity, does one person’s plank length also change compared to another? Then how can it be a constant? So many questions.
Gravity is a reaction like inertia. If 2 rock in series on a string swinging in a circle the inner if forced into the outer determined by the speed of the revolution. The entire solar system is spiralling. Then you have time which is only a measurment from point a to point b ect. You cant go back in time. You have to go back in history or future not time. There is no time fabric, get over it. Also the speed of thought is faster than speed of light. Travling at speed of light 186k mps for a 10 million years or i can think my self there in one second verses 186k mps x 10 million. Now tell me which is faster. I am now going to get on my unicorn and think my self to alpha C. Cya
Well, i dont know why its so hard to figure out that gravity is not what we think it is. Gravity is only one half of the picture. There is still a whole other half of the equation to account for and even then, once u have both halves, the two halves together make a whole other thing. Gravity is a by product of dielectricity and magnatism. When u learn the truth about magnatism and electricity u realize that there actually is no such thing as gravity. Its called something completely different and once u understand that, things start adding up and making a whole lot more sence! No joke! I promise. Einstein knew nothing about electricity and it shows in his work. Where as Nickola Tesla had it all figured out and then some. And for some reason the military has done everything in there power to keep his discoveries to them selves.
Dear Petals, You have interesting comments that gravity is a result of electromagnetism. Please elaborate.
All of you with valued points. Join the dots through time to spots that journey picture shots.
I suggest that Melvin’s suggestion for “knots in the Aether” may be revised.
Such that Planck scale knotting of Space Time (Fabric) be considered to twist up, drawing in vast amounts of Space Time (C²) and creating a stretching of Space Time (fabric) around that point. Thus creating a low density region around this point.
Multiple low density regions, located in an ambient density of Space Time, will be pushed together by the pressure differentials of the caring density.
This seems to imulate the action if gravity.
I suggest low density regions of Space Time will cause refraction of EMF waves (light) as it passes through the region.
I’m suggesting that the smallest point of Matter may be a Planck scale knot in the “Fabric” of Space Time.
Thus Matter = Fabric (Space Time) *C²
If you have read A Brief History of Time, you will encounter the deceased professor Hawkings’ musing about the quest for knowledge about the universe, which i will paraphrase. “We have equations to describe the universe, but they are like bones. What is the fire that informs the equations, that brings them to life ?” I think that being on a”quest” to comprehend the cosmos is the greatest challenge, but I cannot think of a better calling. Professor Hawking wanted to ” know the mind of God”,which is perhaps the oldest philisophical quest.He also mentions that the age of any one person “encompassing” the knowledge of their time is passed;the data is now overwhelming in extent, thanks to newer and newer technology and findings.So how do laymen, and even those in official science, keep a sense of balance?Maybe it is a matter of attitude.We can be humbled before the Mystery,but still enjoy what knowledge we have.And, as Peter Townsend sang,”we can dance while our knowledge is growing !”
Treating Hawking as a god like figure in physics certainly seems to miss the point. Besides, he was far from perfect; insisting at first that Jacob Bekenstein was wrong, only to flip-flop and expand on his theory regarding black hole entropy to lay claim to “Hawking radiation.”
How does gravity work at the particle level? The question has stumped physicists since the two bedrock theories of general relativity (Albert Einstein’s equations envisioning gravity as curves in the geometry of space-time) and quantum mechanics (equations that describe particle interactions) revolutionized the discipline about a century ago.
Right. But LQG is not the solution. It can not explain the absolute/perfect smoothness of space time. SR and GR to be advanced to explain synchronization of QM with GR(from basics of SR)
Some time of “Granular Spacetime” or a universe made of cuboctahedron like packing of spheres will probably give the final theory of everything.
I can’t help wondering if the presence of mass actually causes the curvature of spacetime, or if it’s the curvature of spacetime that results in mass…?
To be or how much to be, that is the question.
Buddha and Christ or for that matter any religious leader probably did not know of the Higgs boson or gravitational waves unless the ancients had some alien visitors with superor intelligence imparting or are still imparting superior knowledge to our feeble minds in ways and technologies unknown yet to us.
The general theory of relativity and quantum mechanics are working models in their realms and we should strive to understand the whole, only a part of which, our evolved senses can perceive. Science by way of mainly mathematics is trying to transcend those very limitations IMHO.
Excellent thought process. Or maybe mass making an impression in time that we perceive to be gravity. If we all take up our own space (mass) in time then it would account for Einstein’s perception theory. It could also explain how and why our universe bends and expands. Space is only 3 dimensions. Why do we have to add to it? Why can’t it be the 4th dimension that’s as real as matter?
I fell asleep and dreamt I was a butterfly…
Good interview but it should be acknowledged that this is not a “new” theory at all. LQG has been around for, what, 20-30 years?
But … a…. without time how can there be any movement, flow a..a.a. any kind of diferrentness or change…. I m not sure if I could even change my mind let alone my undershorts… I’m not sure but maybe we need to work on thus some more as I just don’t think we are quite there yet…
Time is an entangled byproduct of what is really going on, down there, at the quantum level. Causality is the propagation speed of information and massless particles. As one Planck volume does what energy space does, if you can tell us that would be great, it vibrates profusely creating all sorts of fluctuations that then interact with all of the other fluctuations from it’s neighbors. Those interactions cannot be pinned down with a frame of reference, therefore time itself is not the same as sheer existence. In my mind time is not a dimension, so much as it is a handy tool for adding dimensionality to that existing thing. The information interactions are what present the illusion of a forward arrow of time, as they become entangled and change the outcome of different interactions based on their other partner, they sort of create a chain reaction effect. Whatever entanglement really is, and whatever causes there to be a causality speed limit are the important factors. Since wrapping the human mind around things smaller than what we are made of, ie matter, it is quite understandable that it makes no sense. I feel like the Higgs mechanism that gives some interactions mass is kind of the same type of phenomenon. Where everything that has mass is already bound inside of the chain reaction effect, it is born to it or of it I can’t tell. Either way, we can’t not feel like time exists because for all intents and purposes we have mass so it kind of does. I look at it sort of like Schrodinger looked at velocity and position for particles. Where the act of observing a particle means it no longer goes where it might have, and knowing it’s velocity means never knowing the position. But, I ask if that is because there is something fundamentally funny happening down there or because we can never use mass, or particles for that matter, to measure that small. Both really, we can’t ever measure that small so things are mysterious, but that pesky entanglement thing says that particles do spontaneously change. Sometimes location, sometimes spin, but change they do. But they are just the ripples, for lack of a better word, emanating from whatever quantum energy space is. Which is where Schrodinger comes into play. This infinitely fast oscillation of this Planck volume is emanating information faster than we can comprehend. Time means nothing in the ever present now of infinity. In that case, the three spatial dimensions would actually be byproducts as well. The only two things I can think of are that existing thing and entanglement or one of the two is a product of the other. One dimensional, hope all of this helped.
There’s a few theories of geometry and the isotropic nature of quantum physics that are close to making sense of the randomness. But you’re not looking at the bigger picture. What are those vibrations? Why do some see the golden ratio in some of their findings? Could the impression of said matter on the 4th dimensions be the cause of these vibrations? Too small to be felt and seen but everything when it comes to the makeup of the universe? Let’s take a big Rock for example. You throw it in a lake and it makes a huge splash because it’s huge. Dust, would barely make a ripple but it doesn’t mean the lake doesn’t exist. Strangely enough, none of this is new to us. I would love to read more of your opinions though including more of your observations.
I think we need to delve into this question: How much of the universe, perceived by our senses, is a byproduct of our senses themselves? Do we live in a “genetically tailored” universe?
I do believe the biggest mistake modern cosmology makes, is the assumption that we exist separate from an external world.
Excellent article. Interesting theory on how time does not exist at this below subatomic scale. Why is this I wonder? More research funding is needed for these fundamental questions!
Excellent! Creditable!
Can we approach the puzzle of reality the meditation way, the way the Buddha taught ?
In physical world, it is improbable to synchronise Planck and cosmic scales, yet in the arena of consciousness, both the scales are found in sync perfectly.
However large the LHC may be, it may not be the universe-sized and that is when and where reality lies and that can be attained only by way of meditation, i.e; at the level of consciousness.
Ummm… Okay.
If you read over ancient texts, dimensions of life and consciousness might just be different intensities of energy and different levels of molecular structures. We don’t discover anything. We just translate the language of the universe. And the impression of time is exactly that. Impression in time because time is as real if not more so than space itself. Different but intertwined. It can’t be ommited. It expands. Imagine time as water filling up an aquarium. Except it’s more jello like. And read over pythagoras, newton, einstein, and all the ancient scripts all over again. Try and see how gravity would work in that model
In the beginning God created the Heavens and the Earth. Our finite minds are capable of much understanding but are as nothing compared with Almighty God, who not only created everything but sustains it by his Word the Lord Jesus Christ.
Perfect response.
All religions put together are not going to shed one iota of light or any detailed knowledge on theoretical physics. They never have and never will. Neither Christ nor Buddha nor any other major figure of the past from religion has done this. However, if we were to take all the military budgets and monies earmarked to maintain religions for just one year, we might be able to build a decent next generation accelerator. It would provide a lot of jobs, shared purpose and a worthwhile set of goals to strive towards for humanity – as opposed to all the petty disagreements about whose religion or political ideology is right. We need to stop wasting our valuable time/resources building a military apparatus to try to exert our petty ideas/dogmas on each other and prove that each of our petty little groups (or countries) are right.
I will pray for you and for all the Lord’s people who are blinded I love you and he loves you turn to him not to science
That’s a more perfect response. What’s truly amazing about this universe is that human beings are still babbling about religions and ideologies at all.
Read Freud. Pythagoras. All the mathematicians the world had ever produced. Beyond their math, lies the truth in their words. Or better yet. Go stand in front of a hurricane and try to flip dice or pay for your existence. There are literally a billion trillion quadrillion possibilities for you not to exist
In a binary system, it’s as simple as 00. But one perfect algorithm to make all our reality possible. But i forgot. You’re 1000 years old. You know everything there is to know in a universe that’s 4 billion years old. That might be on its billionth cycle
How exactly do we have shared goals and values about what things are worth or not worth without religious, philosophical argument? Even saying that science is “worth” doing at all assumes things about value which would impinge on religion, philosophy, and politics. If science is valuable to humans, it is valuable toward some goal or end for us, but to identify any goals or ends at all is, at minimum, to take a position in a philosophical and theological debate.
Bingo! Religion, and to a lesser extent philosophy, are the only frameworks that allow one to find meaning in existence, and identify the pursuits “worth” doing. Unfortunately that becomes quite problematic when it comes to pursuing scientific knowledge. Religion brings purpose and meaning to ones life at the cost of renouncing reason. The very faculty fundamental to seeking scientific knowledge. While it may seem like one has to choose between reason and religion, it’s never the case. Becoming aware of the choice makes the only possible option impossible to ignore. Once the choice arises, one is stuck with reason and the empty and pointless existence that is made clear. Such is the terrible fate of beings cursed with the terrible and monstrous ability that we call consciousness…
Excellent!!! However, it’s only us humans who complicate things. Religion has been at the forefront of human knowledge and innovation since the dawn of time. Be it for evil profit or self indulgence, the Medici’s once had the world in the palm of their hands using politics and religion. But in doing so, created governments, finance, arts and schools, to name a few. And they are only one of the very few who the world may never know. But they are out there contributing to the pool of human knowledge in muscle memory, life’s SD card in genealogy. Don’t mistake real religion and real spirituality for corruption because unfortunately, like any government, or any type social heirarchy, there is power. And power corrupts. But it can also be the ultimate source of motivation that can change the landscape of human existence time and time again with contributions that can never be quantified. And as many will tell you. What is our science unless God wills us to learn it. There is rhythm in everything around us. All we have to do is listen.
“Ignorance more frequently begets confidence than does knowledge: it is those who know little, and not those who know much, who so positively assert that this or that problem will never be solved by science.” – Charles Darwin
Personally, I find that if there is a creed compatible with our actual reality it’s quite simply to *feel* that everyone and everything matters, but to *know* better than to believe anything about any of it as a matter of faith. It is possible, of course, to discuss, debate, and even investigate the limits of human knowledge. In philosophy this is called epistemology. But it is not helpful for any one person to assume that the limits of their own knowledge are anywhere near anyone, let alone everyone, else’s.
An orchid knows nothing, yet it knows to mimic a bee to survive. I guess natural selection chose it to survive when it had never existed before the bees took to that environment. Some female species of fish evolve into a male to adapt to the harsh reality of the competitive seas. Good thing female fish know how to use calculators. The males are too busy eating or be eaten. Nature, just like our knowledge, adapts to the needs of the world around us. Unless you keep looking up for Eagles tigers and bears because natural selection would have had them wipe out the early humans. But interesting tidbit. Dumb timid lazy prey seem to always outnumber the eat or be eaten mentality. That has to be science also I’m guessing.