The showpiece of the living room in the house I grew up in was an old-fashioned grandfather clock. It was an impressive contraption, ornately filigreed on the outside and intricately mechanical on the inside. My young self would doubtlessly have been even more impressed if he had known that the clock’s functioning depended on conditions established at the beginning of the universe, nearly fourteen billion years ago.
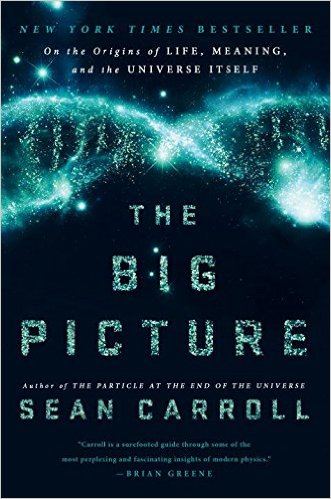
WHAT I LEFT OUT is a recurring feature in which book authors are invited to share anecdotes and narratives that, for whatever reason, did not make it into their final manuscripts. In this installment, theoretical physicist Sean M. Carroll shares a story that didn’t make it into his book “The Big Picture: On the Origins of Life, Meaning, and the Universe Itself.”
The purpose of a clock is to measure the flow of time, from past to present and onto the future. “Time’s arrow” is the name we give to the fact, so obvious as to hardly seem worth remarking on, that the past is different from the future. The past has already happened, while the future remains to be written. We remember what happened yesterday, while the best we can do concerning tomorrow is to make predictions.
Time’s arrow is puzzling — not in its own right, but because of its uneasy relationship with the laws of physics. Ever since Isaac Newton, our best theories of the fundamental, microscopic workings of reality don’t have an arrow of time at all. The equations of physics put the past and future on absolutely equal footing. It’s only when we widen our view to the messy macroscopic world, consisting of enormous collections of particles constantly bumping into each other, that time becomes unbalanced.
The culprit is entropy: the quantity that measures the disorder or randomness of physical systems. A teaspoon of cream and a separate cup of coffee constitute an orderly, low-entropy system; mix them together and the entropy goes up. The Second Law of Thermodynamics tells us that, left to themselves, systems always evolve toward higher entropy rather than lower. Cream and coffee don’t unmix themselves.
To most modern physicists and philosophers, the fact that entropy increases as time passes is the single underlying feature of the world responsible for all of the ways in which the past and future are different. Memories, aging, the tendency of causes to precede effects: all can ultimately be traced to the fact that the entropy of our world was lower yesterday, and will be higher tomorrow.
But the inexorable push of entropy can be avoided in special circumstances. Simple systems, with few moving parts and shielded from the annoyances of noise and dissipation, manifest the temporal egalitarianism of the fundamental laws. A rocking pendulum, in an evacuated chamber and with frictionless bearings, does not exhibit any arrow of time. Its motion looks the same forwards and backwards. It’s more or less a paradigmatic example of evolution without any temporal preference.
That’s fine, as far as it goes. But thinking about it a bit more, we realize there is something puzzling going on. One of the well known uses of pendulums in the real world is to power clocks, like the grandfather clock my family used to own. And if there’s any physical object that relies on the arrow of time, it would be a clock: a while ago the hands were a bit counter-clockwise from where they are now, and a while in the future they will have moved a bit clockwise. Where does that directionality come from, if the pendulum is perfectly reversible? (This example was analyzed by philosopher David Albert, based on discussions by physicists Marian Smoluchowski and Richard Feynman.)
There are two things going on, both of which are crucial to the operation of a pendulum clock. One is a little gizmo called an escapement, which turns the back-and-forth-rocking of the pendulum into the one-way ticking of the clock. Robert Hooke, a rival of Isaac Newton’s, invented the first escapement back in the seventeenth century. The clock hands are driven by an “escape wheel” with pointed teeth that are angled in a uniform direction. The pendulum, meanwhile, is connected to a two-armed piece called the “anchor.” As the anchor rocks back and forth, one of the arms first pushes the escape wheel in one direction, and then the other catches the teeth so that the wheel cannot move in the other. In this way, the oscillations of the pendulum become the uniform motion of the clock hands.
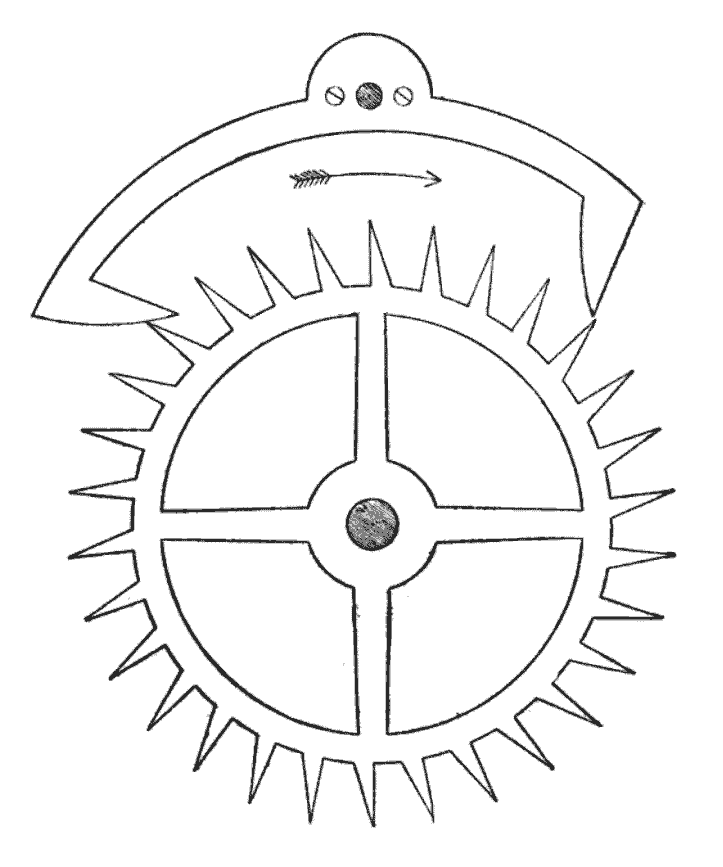
Drawing of an anchor escapement from an 1896 clockmaking manual. (Visual by Wikimedia Commons)
All of this sounds good, and would seem at first to be sufficient: the angling of the anchor arms and the teeth on the escape wheel provide a directionality to the motion of the clock. Except: where did entropy come in? How does the universal arrow of time governed by increasing entropy become related to the local arrow of time of this particular clock?
The answer resides in the seemingly innocent lifting and pushing of the anchor. It seems, by looking at the drawing of an escapement, that the wheel can obviously move in only one direction. But the underlying laws of physics assure us that if something can move in one direction, it can also move in the reversed direction. In this case, that would involve the anchor briefly lifting up, with the escape wheel swiftly and spontaneously moving backwards while it was lifted.
Why doesn’t that happen, and what does it have to do with entropy?
The secret is that clocks tick. That is, they make a small noise at regular intervals. That ticking is precisely the sound of the anchor in the escapement coming into contact with the wheel to drive it forward. It’s this contact that creates the sound we hear, and even — though the effect is so small as to be practically imperceptible — heats up the metal of the escapement just a bit. Both of these effects work to increase the entropy of the universe.
This explains why mechanical pendulum clocks run in one direction and not the other. They could run backwards, under very precise conditions: seemingly random motions in the metal of the escapement could cause it to spontaneously cool down, and sound waves could focus in on the mechanism in precisely the right way to push it backward one step. In order for the clock to move backward for an extended period, this ridiculously unlikely set of influences would have to happen over and over again. It all seems quite silly, not like anything that could happen in the real world.
But this bizarre-sounding sequence (sound waves focus in on the moment when the anchor hits the wheel, which slightly cools down) is what actually does happen (sound waves emanate outward, wheel heats up) only backwards in time. As far as the underlying laws of physics are concerned, either chain of events is perfectly permitted, although to us they seem natural in one direction of time and crazy in the other. The difference is that entropy increases forward in time in the familiar sequence of events, while the time-reversed sequence would require that entropy spontaneously goes down. (We can imagine frictionless, perfectly reversible clocks, but real mechanical ones are noisy, irreversible things.)
The reason why grandfather clocks move in one direction and not the other is that the entropy of the universe is lower in one direction of time and higher in the other, and the noise and heat generated by the clock’s mechanism align with the general trend of increasing entropy. Ultimately, the reason why entropy is lower in the past than in the future comes down to a brute fact: the universe as a whole had an extraordinarily low entropy at its beginning.
Fourteen billion years ago, just after the Big Bang, the matter and radiation that makes up our universe was in an extremely orderly state. Nobody knows why; that’s one of the primary puzzles facing modern cosmology. But given that one cosmic truth, the march of time’s arrow follows very naturally.
When the entropy of a system is as high as it can get, we say that the system is in equilibrium. If a clock and its surrounding environment were in equilibrium, it would be equally likely to move backward as to move forward. In equilibrium, time has no arrow. Thus the passage of time — and the clocks with which we measure it — are echoes of the conditions at the earliest moments in the history of our observable universe.
Sean M. Carroll is a theoretical physicist at Caltech. His current research focus is on the origin of the universe and the arrow of time. He has written several other books, including “From Eternity to Here: The Quest for the Ultimate Theory of Time” and “The Particle at the End of the Universe: How the Hunt for the Higgs Boson Leads Us to the Edge of a New World.”